The Role of Enzymes in Biochemical Reactions: A Comprehensive Guide
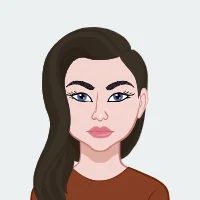
The Role of Enzymes in Biochemical Reactions: A Comprehensive Guide is a comprehensive resource that delves into the vital role enzymes play in biochemical reactions. Authored by leading experts in the field, this guide offers a detailed exploration of enzyme structure, function, kinetics, and regulation, providing a thorough understanding of their significance in biological processes.
Enzymes are biological catalysts that facilitate chemical reactions within living organisms by lowering the activation energy required for these reactions to occur. This guide elucidates the mechanisms through which enzymes accelerate reactions, highlighting key concepts such as enzyme-substrate specificity and the induced fit model. By elucidating the complex interplay between enzymes and substrates, the guide offers insights into how enzymes recognize and bind to specific substrates, initiating and facilitating chemical transformations.
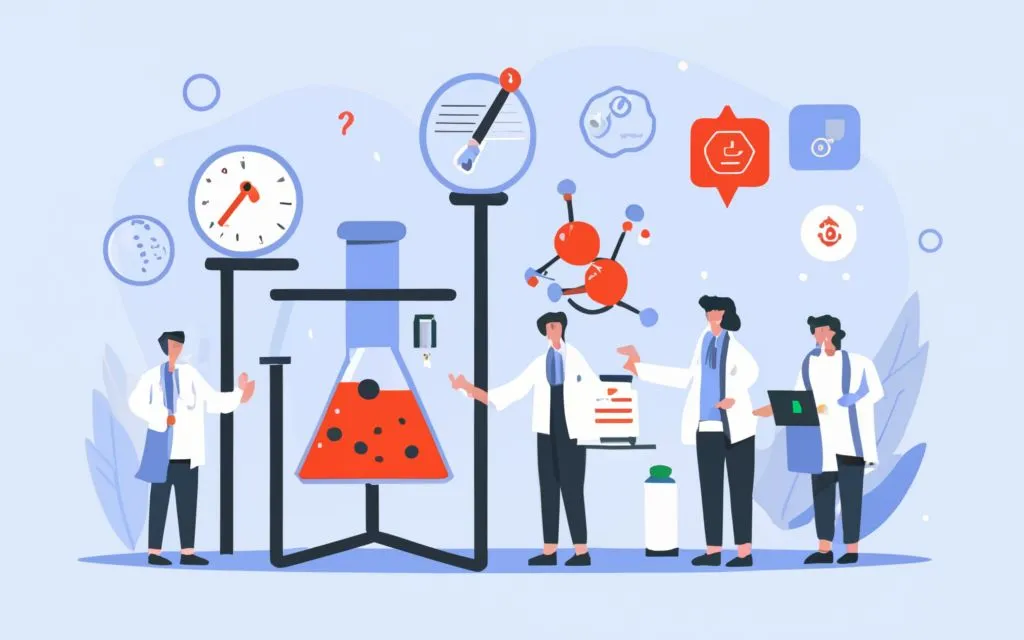
Furthermore, the guide discusses enzyme kinetics, including the Michaelis-Menten equation and its application in determining enzyme kinetics parameters such as the maximum reaction rate (Vmax) and the Michaelis constant (Km). Understanding these kinetics parameters is essential for elucidating the catalytic efficiency of enzymes and their substrate-binding affinity.
Moreover, the guide delves into enzyme regulation, elucidating the various mechanisms through which enzyme activity is modulated to meet the dynamic needs of the cell. This includes allosteric regulation, covalent modification, and the influence of factors such as pH, temperature, and substrate concentration on enzyme activity.
The guide also explores the diverse roles of enzymes in metabolism, highlighting their involvement in essential pathways such as glycolysis, the citric acid cycle, and oxidative phosphorylation. By elucidating the specific enzymes involved in each metabolic pathway and their respective functions, the guide provides a comprehensive overview of how enzymes drive cellular metabolism.
Additionally, the guide discusses the applications of enzymes in biotechnology, including their use as biocatalysts in industrial processes and their significance in fields such as medicine and agriculture. From the production of pharmaceuticals to the enhancement of crop yields, enzymes play a crucial role in a wide array of applications that benefit society.
Understanding Enzymes and Their Functionality
Enzymes are biological molecules that act as catalysts, speeding up chemical reactions within living organisms without being consumed or permanently altered in the process. They are crucial for numerous physiological processes, including metabolism, digestion, and cellular signaling. Understanding enzymes and their functionality is fundamental to grasping the intricacies of biochemical processes and their significance in various biological systems.
Enzymes possess specific structural features that enable them to facilitate reactions efficiently. Their active sites, regions where substrates bind and undergo chemical transformation, exhibit high specificity for particular substrates. This specificity arises from the precise arrangement of amino acid residues within the enzyme's structure, creating a complementary fit for the substrate.
Enzyme functionality relies on several key principles. Firstly, enzymes lower the activation energy required for a reaction to occur, thereby accelerating the rate of the reaction. This reduction in activation energy occurs by stabilizing the transition state of the reaction, facilitating the conversion of substrates into products.
Moreover, enzymes exhibit optimal activity under specific conditions, including pH, temperature, and substrate concentration. Deviations from these optimal conditions can affect enzyme activity and may lead to denaturation, where the enzyme loses its structure and function.
Enzyme activity can be regulated through various mechanisms to ensure precise control of biochemical pathways. Regulatory molecules, such as activators and inhibitors, modulate enzyme activity by altering the enzyme's conformation or accessibility to substrates. Additionally, allosteric regulation involves the binding of molecules at sites other than the active site, inducing conformational changes that affect enzyme activity.
Enzymes participate in a wide range of biochemical reactions, including metabolic pathways involved in energy production, biosynthesis of essential molecules, and degradation of substrates. For instance, digestive enzymes like amylase, protease, and lipase break down carbohydrates, proteins, and lipids into smaller molecules that can be absorbed and utilized by the body.
What are Enzymes?
Enzymes are proteins that act as biological catalysts, speeding up the rate of chemical reactions without being consumed in the process. They lower the activation energy required for a reaction to occur, making it easier for substrates to undergo transformation into products. Enzymes are highly specific, recognizing and binding to particular substrates based on their complementary shapes, much like a lock and key.
Enzyme Structure and Classification
Enzymes exhibit complex three-dimensional structures that are crucial for their functionality. The structure of an enzyme determines its active site, the region where substrate binding and catalysis occur. Enzymes can be classified into several categories based on their mode of action, including oxidoreductases, transferases, hydrolases, lyases, isomerases, and ligases.
Mechanisms of Enzyme Action
Enzyme catalysis involves several key steps, including substrate binding, catalytic reaction, and product release. The active site of an enzyme provides a microenvironment that facilitates specific chemical reactions, such as acid-base catalysis, covalent catalysis, and metal ion catalysis. Enzymes can also undergo conformational changes upon substrate binding, further enhancing their catalytic efficiency.
Factors Affecting Enzyme Activity
The activity of enzymes is influenced by various factors, including temperature, pH, substrate concentration, and the presence of inhibitors or activators. Enzymes exhibit optimal activity within a specific temperature and pH range, beyond which their activity may decline due to denaturation. Additionally, changes in substrate concentration can affect the rate of enzyme-catalyzed reactions through processes such as substrate saturation and competitive inhibition.
Enzyme Kinetics: Exploring Reaction Rates and Kinetic Parameters
Enzyme kinetics is a branch of biochemistry that focuses on studying the rates of enzyme-catalyzed reactions and the factors that influence them. Enzymes are biological catalysts that accelerate chemical reactions by lowering the activation energy required for the reaction to occur, thus speeding up the rate of the reaction without being consumed in the process.
The study of enzyme kinetics involves understanding the relationship between the concentration of substrates and products over time and how this relates to the enzyme's catalytic activity. This field is crucial for understanding the mechanisms of enzyme action and for various practical applications in fields such as medicine, agriculture, and industry.
One of the key concepts in enzyme kinetics is the Michaelis-Menten equation, which describes the relationship between the initial reaction rate (v₀), the maximum reaction rate (Vmax), the substrate concentration ([S]), and the Michaelis constant (Km). This equation is essential for determining important kinetic parameters such as Vmax and Km, which provide insights into the efficiency and specificity of an enzyme.
Experimental techniques used in enzyme kinetics include spectrophotometry, which measures changes in absorbance of light as a reaction progresses, and chromatography, which separates and quantifies reaction components. These techniques allow researchers to determine reaction rates under various conditions and to study factors such as pH, temperature, and enzyme or substrate concentration that affect enzyme activity.
Enzyme inhibition is another important aspect of enzyme kinetics. Inhibitors can be classified as reversible or irreversible and competitive, non-competitive, or uncompetitive, depending on their mode of action. Understanding enzyme inhibition is crucial for drug discovery and development, as many drugs act by inhibiting specific enzymes involved in disease processes.
The study of enzyme kinetics has practical applications in various fields. In medicine, it is essential for understanding drug metabolism and designing effective therapeutic agents. In agriculture, enzyme kinetics can be applied to improve crop yields and develop pest control strategies. In industry, it is used in the production of food, beverages, and biofuels, as well as in waste treatment processes.
Enzyme Kinetics Overview
Enzyme kinetics is the study of the rates of enzyme-catalyzed reactions and the factors that influence these rates. Kinetic analysis allows for the determination of important parameters such as reaction rate constants, Michaelis constant (Km), and maximum reaction velocity (Vmax), providing insights into the catalytic efficiency and substrate specificity of enzymes.
Michaelis-Menten Kinetics
The Michaelis-Menten equation describes the relationship between substrate concentration and reaction rate in enzyme-catalyzed reactions. This equation, derived from the Michaelis-Menten kinetic model, helps elucidate the kinetic parameters Km and Vmax, which are crucial for understanding enzyme-substrate interactions and catalytic efficiency.
Lineweaver-Burk Plot
The Lineweaver-Burk plot, also known as the double reciprocal plot, is a graphical representation of the Michaelis-Menten equation. This plot allows for the linearization of enzyme kinetics data, making it easier to determine kinetic parameters such as Km and Vmax through graphical analysis. The Lineweaver-Burk plot is a valuable tool for studying enzyme inhibition and comparing the kinetic properties of different enzymes.
Enzyme Inhibition
Enzyme inhibition refers to the process by which the activity of an enzyme is hindered or suppressed by the presence of inhibitors. Inhibitors can be classified as reversible or irreversible based on the nature of their interaction with the enzyme. Common types of reversible inhibition include competitive, noncompetitive, and uncompetitive inhibition, each of which affects enzyme kinetics differently and can have important implications in drug development and enzyme regulation.
Applications of Enzymes in Various Fields
Enzymes, often described as nature's remarkable catalysts, are biological molecules renowned for their ability to accelerate chemical reactions within living organisms with exceptional specificity and efficiency. These versatile molecules have found extensive applications across a myriad of industries, ranging from food and beverage to healthcare, textile, detergent, pharmaceuticals, bioremediation, biofuels, and biotechnology. Their pivotal role in enhancing processes, improving production efficiency, and driving innovation has revolutionized numerous sectors, shaping modern industrial practices and promoting sustainability. This article provides a comprehensive overview of the diverse applications of enzymes in various fields, highlighting their invaluable contributions to scientific research, industrial production, and environmental sustainability.
Enzymes in Food Industry
Enzymes play crucial roles in food processing and production, where they are used to enhance flavor, texture, and nutritional value, as well as to improve process efficiency. Examples include the use of proteases in cheese production, amylases in bread making, and carbohydrases in fruit juice clarification.
Enzymes in Medicine and Healthcare
Enzymes are widely utilized in medicine and healthcare for diagnostic, therapeutic, and research purposes. They are employed in clinical assays for biomarker detection, as therapeutic agents for enzyme replacement therapy, and as tools for studying biochemical pathways and disease mechanisms.
Enzymes in Biotechnology
Enzymes are integral to numerous biotechnological applications, including recombinant DNA technology, protein engineering, and biocatalysis. They are used in the production of biofuels, pharmaceuticals, and biodegradable polymers, as well as in the synthesis of fine chemicals and pharmaceutical intermediates.
Enzymes in Environmental Remediation
Enzymes play a vital role in environmental remediation efforts aimed at mitigating pollution and waste. Enzymatic processes are employed in wastewater treatment, bioremediation of contaminated soil and water, and the degradation of pollutants such as pesticides, hydrocarbons, and industrial chemicals, offering sustainable solutions to environmental challenges.
Conclusion
In conclusion, enzymes stand as the cornerstone of biochemical reactions, orchestrating the intricacies of cellular processes with remarkable precision and efficiency. Their diverse roles span across metabolic pathways, signal transduction, DNA replication, and beyond, underscoring their indispensability in sustaining life processes. Through this comprehensive guide, we have explored the fundamental principles governing enzyme kinetics, substrate specificity, and regulation, shedding light on the intricate mechanisms underlying enzymatic activity.
Moreover, we have delved into the practical applications of enzymes across various industries, from food and beverage to pharmaceuticals and biotechnology, showcasing their pivotal role in enhancing production processes, driving innovation, and promoting sustainability. Enzymes continue to revolutionize industrial practices, offering greener alternatives and unlocking new possibilities in bioremediation, biofuels production, and healthcare.
As our understanding of enzymes deepens, fueled by ongoing research and technological advancements, their potential to address pressing global challenges becomes increasingly evident. From combating environmental pollution to advancing personalized medicine, enzymes hold promise in addressing multifaceted societal needs.