The Visual System and Retina: Exploring Anatomy, Physiology, and Perception
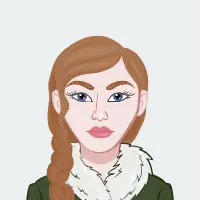
The human visual system represents a remarkable feat of biological evolution, finely tuned to capture and process visual information from the environment. Central to this intricate mechanism is the retina, a specialized neural tissue lining the back of the eye. Here, light undergoes a complex transformation into neural signals through the photoreceptor cells—rods for low-light vision and cones for color perception. These signals are then processed by layers of bipolar and ganglion cells before being transmitted via the optic nerve to the brain. Understanding the structure and function of the retina and its role in visual processing will provide a solid foundation for your studies. This exploration will also serve as a valuable resource for your biology assignment, offering insights into the intricate workings of the human visual system.
Anatomy-wise, the retina consists of distinct layers, each with specific roles in visual processing. Physiology-wise, the retina's photoreceptors initiate the crucial conversion of light into electrical signals, setting off a chain reaction that culminates in visual perception. Beyond the retina, higher cortical areas such as the lateral geniculate nucleus and the striate cortex play pivotal roles in further processing and interpreting visual information.
Understanding these intricate anatomical structures, physiological processes, and perceptual mechanisms is crucial for comprehending how we perceive the world visually. This blog explores these components in-depth, highlighting their integration and collaboration within the visual system to provide us with the rich and nuanced experience of sight.
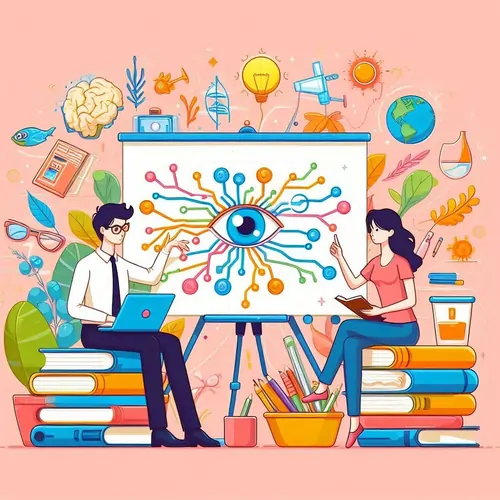
Anatomy and Physiology of the Retina
The retina, positioned at the rear of the eye, plays a pivotal role in the intricate process of visual perception. Comprised of multiple layers, it is structured to efficiently capture and process light signals. Photoreceptors within the retina are specialized cells that detect light: rods are sensitive to low-light conditions, facilitating night vision, while cones distinguish colors under normal lighting. These photoreceptors initiate the conversion of light into neural signals, marking the beginning of visual processing.
Subsequently, bipolar cells transmit these signals to ganglion cells, which compile and transmit the processed visual information through their axons, collectively forming the optic nerve. This nerve serves as a direct conduit to the brain, where visual stimuli are further analyzed and interpreted.
The intricate cellular architecture and functions of these retinal layers are crucial in deciphering how visual information is received, processed, and eventually interpreted by the brain. Understanding these fundamental mechanisms not only sheds light on the complexities of human vision but also informs advancements in ophthalmology and visual neuroscience, potentially leading to innovations in treating visual impairments and enhancing overall visual perception.
The Lateral Geniculate Nucleus (LGN)
The lateral geniculate nucleus (LGN), nestled within the thalamus, plays a crucial role as a relay center in the visual pathway, transmitting processed visual information from the retina to the primary visual cortex. Its organization into distinct layers and intricate network of neurons form receptive fields that respond to specific visual stimuli characteristics such as orientation, spatial frequency, and contrast.
Each of the six layers in the LGN receives input from different types of retinal ganglion cells, segregating information into channels dedicated to conveying particular aspects of visual data. This segregation is essential for processing complex visual scenes effectively. Moreover, the LGN exhibits a unique pattern of connectivity with various cortical areas, facilitating the integration of visual information across different modalities and dimensions.
The receptive field properties of LGN cells, including their size, shape, and responsiveness to light stimuli, further refine visual signals before they reach higher cortical areas. This preprocessing enhances the precision and specificity of visual information processing, laying the groundwork for sophisticated visual perception tasks such as object recognition and motion detection.
In essence, by studying the distinctive characteristics and functional contributions of LGN cells, researchers deepen their understanding of how early visual processing shapes perceptual experiences beyond the retina. This knowledge not only elucidates fundamental principles of sensory processing but also informs advancements in fields like neurology and ophthalmology, aiming to enhance diagnostics and treatments for visual disorders.
The Striate Cortex: Primary Visual Cortex
The striate cortex, also referred to as V1, represents a critical hub where the intricate process of visual processing initiates. Comprising a dense network of neurons, V1 is meticulously organized into functional columns and layers, each contributing uniquely to visual perception. These neurons form receptive fields—specific regions that respond selectively to particular visual stimuli such as edges, colors, and orientations.
Cytoarchitecture within V1 underscores its complex cellular composition, where distinct types of neurons are strategically arranged to optimize the detection and integration of visual information. This organizational structure enables the cortex to parse incoming visual signals with remarkable precision, facilitating the perception of detailed features and spatial relationships within the visual field.
Through the study of V1's receptive field organization, researchers gain profound insights into how the brain deciphers basic visual attributes. Neuronal responses within these fields are finely tuned to detect specific patterns and contrasts, thereby enabling the synthesis of coherent visual percepts essential for recognizing objects and scenes.
In summary, the striate cortex serves as a foundational platform for visual cognition, where the interplay of neural architecture and receptive field properties intricately supports the brain's ability to interpret and make sense of the visual world.
Extrastriate Areas and Higher Visual Processing
Beyond V1, which serves as the primary visual cortex, the brain's extrastriate areas like V2, V3, and V4 play critical roles in processing intricate visual information. These areas exhibit distinct receptive field properties tailored to detect specific visual features such as shapes, colors, and motion directions. V2, for instance, integrates inputs from V1 and contributes to forming more complex visual representations. V3 is specialized in processing motion, enabling the brain to perceive and track moving objects accurately. V4 is crucial for color perception, enhancing our ability to differentiate hues and shades.
What sets these extrastriate areas apart is their interconnectedness with other brain regions involved in higher-order cognitive functions. They collaborate extensively with areas responsible for memory, attention, and decision-making, thereby influencing our perception of spatial relationships, object recognition, and even emotional responses triggered by visual stimuli. By understanding these specialized neural networks, researchers gain insights into how the brain constructs coherent visual experiences from fragmented sensory inputs. This knowledge not only enhances our comprehension of visual processing mechanisms but also holds promise for developing therapies and technologies aimed at restoring or enhancing visual abilities in clinical settings.
ON and OFF Channels, and Brightness Perception
The distinction between ON and OFF channels within the visual system plays a crucial role in encoding contrasts and perceiving brightness. These pathways enable the visual system to distinguish between light increments (ON) and decrements (OFF), influencing our perception of light intensity and contrast in the visual environment.
Midget and Parasol Systems: Specialized Pathways for Visual Information
The midget and parasol systems are specialized pathways within the retina that handle specific aspects of visual information. The midget system, characterized by small receptive fields and high visual acuity, is essential for color vision and fine detail. In contrast, the parasol system, with larger receptive fields and high sensitivity to motion, contributes to detecting movement and changes in the visual scene.
Color Vision, Adaptation, and Visual Perception
Color vision relies on the processing of different wavelengths of light by specialized cone cells in the retina. The brain integrates this information to perceive a spectrum of colors, while adaptation mechanisms adjust our visual sensitivity to varying light conditions. Exploring these processes deepens our understanding of how the visual system adapts to changes in the environment and sustains accurate color perception.
Eye Movement Control: From Subcortical to Cortical Structures
Controlling eye movements involves intricate coordination between subcortical structures (e.g., brainstem and cerebellum) and cortical areas (e.g., frontal eye fields and parietal cortex). Subcortical structures generate and regulate basic eye movements, while cortical areas refine these movements based on visual input and cognitive processes. This dual pathway system ensures precise gaze control and visual tracking essential for interacting with our surroundings.
Motion Perception and Smooth-Pursuit Eye Movements
Motion perception encompasses how the visual system detects, processes, and interprets moving stimuli. Cortical and subcortical visual structures collaborate to analyze motion direction, speed, and trajectory, facilitating behaviors such as pursuit eye movements. These movements allow us to smoothly track moving objects across our visual field, enhancing our ability to interact with dynamic environments.
Depth Perception, Pattern Recognition, and Visual Illusions
Depth perception enables us to perceive spatial relationships and distances between objects in our surroundings. Neural mechanisms, including binocular and monocular cues, contribute to depth perception, enriching our visual experience. Pattern recognition further enhances our ability to discern shapes and structures, highlighting the brain's capacity for organizing visual information into meaningful forms.
Visual illusions, such as the Müller-Lyer illusion and the Ponzo illusion, provide valuable insights into how the brain processes visual information. By studying these perceptual phenomena, researchers uncover underlying neural mechanisms and principles of visual cognition, advancing our understanding of how perception can be influenced by contextual cues and geometric relationships.
Visual Prosthesis: Innovations in Restoring Vision
Recent advancements in visual prosthesis aim to restore vision for individuals with visual impairments by integrating electronic devices directly with the visual system. These innovative prostheses circumvent damaged retinal cells or optic nerves, transmitting visual information directly to the brain. Current research on visual prostheses focuses on enhancing their effectiveness and reliability, addressing challenges such as achieving precise visual perception and ensuring long-term device functionality. Ongoing efforts in this field aim to improve visual function significantly, offering promising solutions for individuals affected by conditions like retinitis pigmentosa and age-related macular degeneration. Ultimately, the development of advanced visual prostheses holds the potential to not only restore but also enhance visual capabilities, thereby enhancing quality of life and independence for those with visual impairments.
Conclusion:
In conclusion, the study of the visual system and retina unveils the intricate mechanisms underlying human vision. From the initial capture of light by photoreceptors in the retina to the sophisticated processing of visual information in cortical areas, each stage contributes to our ability to perceive and interact with the world. By comprehensively exploring these topics, we not only deepen our understanding of visual neuroscience but also pave the way for future innovations in vision research and clinical applications. As we continue to unravel the complexities of visual perception, our appreciation for the remarkable capabilities of the human visual system grows, illuminating new pathways for exploration and discovery.