Understanding DNA Replication: A Student’s Guide
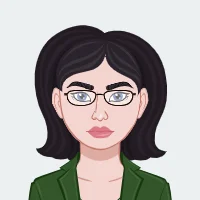
DNA replication is a fundamental process that occurs within every living organism, serving as the basis for genetic inheritance and the transmission of traits from one generation to the next. For students embarking on a journey to comprehend the intricacies of molecular biology, grasping the mechanics of DNA replication is paramount. This blog aims to provide a concise yet comprehensive overview of DNA replication, tailored specifically to students seeking to deepen their understanding of this fundamental biological process. Understanding DNA replication is essential for mastering the foundational concepts of molecular biology and genetic inheritance, enabling students to analyze and interpret biological phenomena at the molecular level with precision and accuracy.
At its core, DNA replication is the process by which a cell duplicates its DNA in order to generate two identical copies, ensuring the accurate transmission of genetic information during cell division. This intricate process involves a series of coordinated steps orchestrated by a multitude of enzymes and proteins, each playing a crucial role in maintaining the integrity of the genetic material.
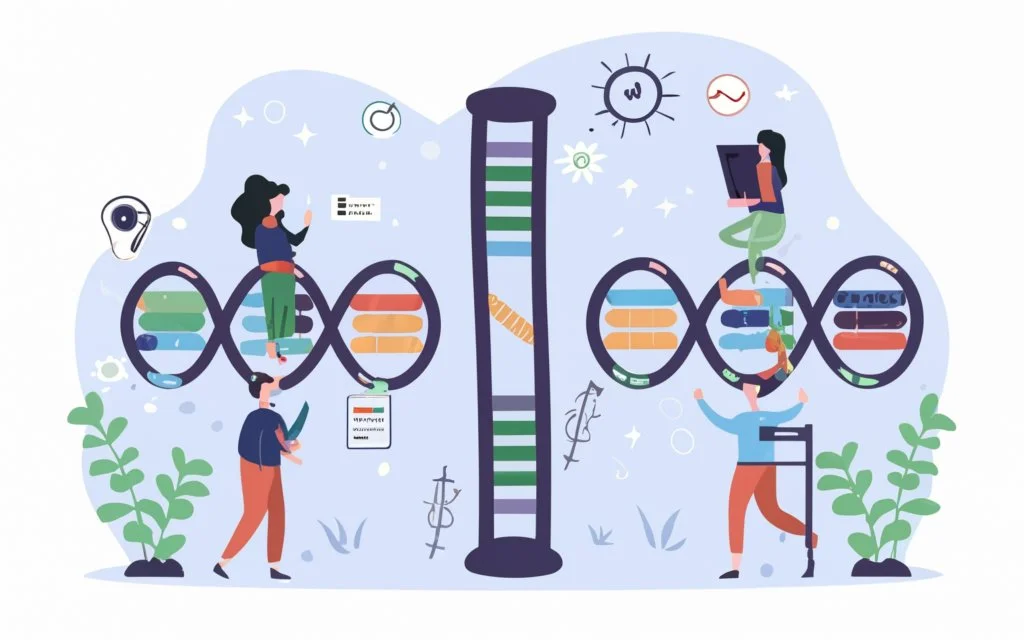
The process of DNA replication can be divided into several key stages, beginning with the unwinding of the DNA double helix by the enzyme helicase. This unwinding creates two separate strands, each of which serves as a template for the synthesis of a new complementary strand. The enzyme DNA polymerase then facilitates the addition of nucleotides to the growing strand, ensuring that the new DNA molecule is an exact replica of the original.
In addition to DNA polymerase, other enzymes such as primase and ligase are also involved in the replication process. Primase synthesizes short RNA primers that provide a starting point for DNA synthesis, while ligase seals the gaps between newly synthesized DNA fragments, ensuring that the two strands are seamlessly joined together.
The process of DNA replication is highly accurate, thanks in part to the proofreading capabilities of DNA polymerase, which helps to detect and correct any errors that may arise during synthesis. However, despite these mechanisms, occasional errors known as mutations can still occur, contributing to genetic diversity and evolution.
Furthermore, DNA replication is a highly regulated process, with checkpoints and control mechanisms ensuring that each step occurs in the correct sequence and at the appropriate time. These checkpoints help to prevent errors and ensure the faithful transmission of genetic information from one generation to the next.
Understanding the process of DNA replication is not only crucial for students studying molecular biology but also provides valuable insights into the mechanisms underlying various genetic disorders and diseases. By unraveling the intricacies of DNA replication, students can gain a deeper appreciation for the molecular basis of life and the remarkable complexity of the biological world.
The Basics of DNA Replication
DNA replication is a fundamental process in all living organisms, essential for the accurate transmission of genetic information from one generation to the next. At its core, DNA replication involves the duplication of a cell's entire genome, ensuring that each daughter cell receives an identical copy of the genetic material during cell division.
The process of DNA replication can be broken down into several key steps. Firstly, the double-stranded DNA molecule unwinds with the help of the enzyme helicase, creating two separate strands. These strands then serve as templates for the synthesis of new complementary strands.
Next, the enzyme DNA polymerase adds nucleotides to the growing strands, following the principle of base pairing: adenine (A) pairs with thymine (T), and cytosine (C) pairs with guanine (G). This ensures that the newly synthesized DNA molecules are identical to the original strands.
In addition to DNA polymerase, other enzymes such as primase and ligase play crucial roles in the replication process. Primase synthesizes short RNA primers that provide a starting point for DNA synthesis, while ligase seals the gaps between newly synthesized DNA fragments, ensuring the continuity of the DNA strand.
DNA replication is a highly accurate process, with mechanisms in place to detect and correct errors that may arise during synthesis. DNA polymerase possesses proofreading capabilities, enabling it to remove mismatched nucleotides and replace them with the correct ones.
Furthermore, DNA replication is tightly regulated to ensure that each step occurs in the correct sequence and at the appropriate time. Checkpoints and control mechanisms monitor the process, helping to prevent errors and maintain the integrity of the genetic material.
Understanding the basics of DNA replication is crucial for students studying molecular biology, as it provides insights into the molecular mechanisms underlying genetic inheritance and cellular processes. By grasping the fundamental principles of DNA replication, students can gain a deeper appreciation for the complexity of life at the molecular level.
The Structure of DNA
Before delving into DNA replication, it's essential to understand the structure of DNA itself. DNA, or deoxyribonucleic acid, is a double-stranded molecule that resembles a twisted ladder, known as the double helix. Each strand is composed of nucleotides, which consist of a sugar (deoxyribose), a phosphate group, and one of four nitrogenous bases: adenine (A), thymine (T), cytosine (C), and guanine (G). The two strands of DNA are complementary, meaning that the sequence of bases on one strand determines the sequence on the other.
The Importance of DNA Replication
DNA replication is essential for various biological processes, including cell division, growth, and repair. During cell division, each daughter cell must receive an identical copy of the genetic material to ensure genetic continuity. Additionally, DNA replication plays a crucial role in DNA repair mechanisms, ensuring the maintenance of genomic integrity.
Key Players in DNA Replication
Several key players are involved in the process of DNA replication. These include DNA polymerase, the enzyme responsible for catalyzing the synthesis of new DNA strands, as well as various accessory proteins and enzymes that aid in unwinding the DNA double helix and stabilizing the replication machinery.
The Steps of DNA Replication
DNA replication occurs in a highly coordinated and regulated manner, involving several distinct steps. These steps include initiation, during which the replication machinery assembles at specific sites on the DNA molecule; elongation, where new DNA strands are synthesized using existing strands as templates; and termination, marking the completion of the replication process.
Mechanisms of DNA Replication
DNA replication is a vital process that ensures the faithful transmission of genetic information from one generation to the next. The mechanisms underlying DNA replication involve a series of coordinated steps orchestrated by a multitude of enzymes and proteins, each playing a crucial role in maintaining the integrity of the genetic material.
At the heart of DNA replication lies the unwinding of the DNA double helix, facilitated by the enzyme helicase. This unwinding creates two separate strands, known as the leading and lagging strands, which serve as templates for the synthesis of new complementary strands.
The synthesis of new DNA strands is carried out by the enzyme DNA polymerase, which adds nucleotides to the growing strand in a 5' to 3' direction. The leading strand is synthesized continuously in the same direction as the replication fork, while the lagging strand is synthesized discontinuously in short segments called Okazaki fragments.
Primase, another key enzyme involved in DNA replication, synthesizes short RNA primers that provide a starting point for DNA synthesis by DNA polymerase. These primers are later replaced by DNA nucleotides, resulting in the formation of a continuous DNA strand.
Once the new DNA strands have been synthesized, they must be joined together to form a complete double helix. This task is accomplished by the enzyme ligase, which seals the gaps between the Okazaki fragments on the lagging strand, ensuring that the two strands are seamlessly joined together.
Throughout the process of DNA replication, various checkpoints and control mechanisms ensure the accuracy and fidelity of the process. These checkpoints help to detect and repair any errors that may arise during replication, ensuring that the genetic information is faithfully transmitted from one generation to the next.
Semiconservative Replication
DNA replication follows a semiconservative model, where each daughter DNA molecule contains one parental strand and one newly synthesized strand. This model was first proposed by Watson and Crick and has since been experimentally validated.
DNA Polymerase: The Workhorse Enzyme
DNA polymerase is the enzyme responsible for catalyzing the addition of nucleotides to the growing DNA strand during replication. It plays a central role in DNA replication and is highly processive, meaning that it can catalyze the addition of thousands of nucleotides without dissociating from the DNA template.
Leading and Lagging Strands
During DNA replication, the two strands of the double helix are unwound, resulting in the formation of a leading strand and a lagging strand. The leading strand is synthesized continuously in the 5' to 3' direction, while the lagging strand is synthesized discontinuously in the form of short fragments known as Okazaki fragments.
Okazaki Fragments: Stitching the Lagging Strand Together
Okazaki fragments are short DNA fragments synthesized on the lagging strand during DNA replication. These fragments are later joined together by DNA ligase to form a continuous strand. This process ensures the accurate and efficient replication of the lagging strand.
Regulation and Errors in DNA Replication
DNA replication is a meticulously regulated process that ensures the faithful transmission of genetic information from one generation to the next. However, despite its precision, errors can occasionally occur, leading to mutations that may have profound consequences for an organism. Understanding the regulation and potential errors in DNA replication is crucial for comprehending the mechanisms underlying genetic diversity and disease.
Regulation of DNA replication involves a complex interplay of various enzymes, proteins, and regulatory factors that coordinate the timing and fidelity of the replication process. Key regulatory mechanisms include the activation of replication origins, which serve as starting points for DNA synthesis, and the recruitment of replication factors that facilitate the unwinding of the DNA double helix and the synthesis of new DNA strands.
Additionally, the cell cycle serves as a fundamental regulatory framework for DNA replication, ensuring that replication occurs at the appropriate time and in coordination with other cellular processes. Checkpoints within the cell cycle monitor the progression of DNA replication, allowing for the detection and correction of errors before they are perpetuated in subsequent generations.
Despite the stringent regulatory mechanisms in place, errors in DNA replication can still occur, leading to mutations that alter the genetic code. These errors can arise from various sources, including spontaneous chemical changes in the DNA molecule, exposure to mutagenic agents such as UV radiation or certain chemicals, and mistakes made by the DNA replication machinery itself.
The consequences of DNA replication errors can vary widely, ranging from benign mutations with no discernible effect to severe genetic disorders and diseases. Some mutations may confer a selective advantage, leading to evolutionary adaptations, while others may disrupt essential cellular functions, contributing to conditions such as cancer or genetic syndromes.
Furthermore, certain genetic disorders, known as DNA replication syndromes, are characterized by defects in the DNA replication machinery itself, leading to a predisposition to replication errors and an increased risk of disease. Understanding the molecular mechanisms underlying these syndromes is crucial for developing targeted therapeutic strategies and interventions.
Ensuring Accuracy: Proofreading and Repair Mechanisms
Despite the remarkable fidelity of DNA replication, errors can still occur. To maintain genomic integrity, cells have evolved proofreading and repair mechanisms to correct errors that arise during replication. These mechanisms include the activity of DNA polymerase, as well as specialized repair enzymes such as DNA mismatch repair and nucleotide excision repair.
Telomeres: Protective Caps of DNA
Telomeres are specialized structures located at the ends of linear chromosomes. They consist of repetitive DNA sequences and play a crucial role in protecting the integrity of the chromosome ends during DNA replication. Telomeres undergo shortening with each round of cell division, eventually leading to cellular senescence or apoptosis.
DNA Replication Errors: Causes and Consequences
DNA replication errors can arise due to various factors, including the spontaneous hydrolysis of nucleotide bases, exposure to mutagens, or errors introduced during DNA synthesis. These errors can have significant consequences, ranging from benign mutations to severe genetic disorders and cancer.
Regulation of DNA Replication: Timing and Control Mechanisms
DNA replication is tightly regulated to ensure its accurate and timely completion. This regulation involves the coordination of various cellular processes, including cell cycle checkpoints, the availability of replication factors, and the activity of regulatory proteins such as cyclins and cyclin-dependent kinases (CDKs).
Conclusion
In conclusion, DNA replication is a fundamental process that ensures the faithful transmission of genetic information from one generation to the next. By understanding the basics, mechanisms, and regulation of DNA replication, students can gain valuable insights into the intricate workings of molecular biology and appreciate the significance of this essential biological process.
DNA replication is a complex and highly regulated process that is essential for the faithful transmission of genetic information from one generation to the next. In this comprehensive guide, we have explored the basics, mechanisms, and regulation of DNA replication, providing students with a thorough understanding of this fundamental biological process. By delving into the structure of DNA, the importance of DNA replication, key players involved, and the steps of DNA replication, students can gain a solid foundation in molecular biology.
The semiconservative replication model, proposed by Watson and Crick, highlights the importance of accurately copying the genetic material during cell division. DNA polymerase, the workhorse enzyme of DNA replication, plays a central role in synthesizing new DNA strands and ensuring the fidelity of the replication process. Understanding the dynamics of leading and lagging strands, as well as the synthesis and processing of Okazaki fragments, provides insight into the intricacies of DNA replication.
Regulation mechanisms ensure that DNA replication occurs accurately and at the right time during the cell cycle. Cell cycle checkpoints, replication factors, and regulatory proteins such as cyclins and cyclin-dependent kinases (CDKs) coordinate the replication process and prevent errors. Despite these mechanisms, DNA replication errors can still occur, leading to mutations and genetic disorders. Proofreading and repair mechanisms, including DNA mismatch repair and nucleotide excision repair, help maintain genomic integrity.
Telomeres, protective caps of DNA at the ends of linear chromosomes, play a crucial role in preserving chromosome stability during DNA replication. However, telomeres undergo shortening with each cell division, eventually leading to cellular senescence or apoptosis. Understanding the role of telomeres provides insight into aging and cancer.