Genetics Problem Solving: A Step-by-Step Guide for Biology Assignments
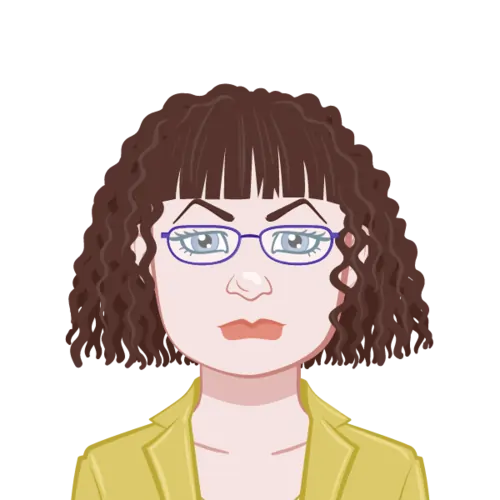
Genetics Problem Solving: A Step-by-Step Guide for Biology Assignments< serves as a comprehensive resource for biology students, providing them with practical guidance on how to approach and solve genetics-related problems commonly encountered in assignments and coursework. This guide is designed to aid both beginners and those looking to deepen their understanding of genetics concepts and their application in problem-solving scenarios. If you need assistance with your Genetics Assignment, this guide offers step-by-step instructions and examples to help you tackle complex problems with confidence.
The blog begins by emphasizing the importance of understanding foundational concepts in genetics. It highlights key topics such as Mendelian inheritance patterns, chromosome structure, and genetic variation principles. By grasping these fundamental concepts, students are better equipped to interpret genetic information accurately and tackle more complex problems with confidence.
A systematic problem-solving approach is then outlined in the blog, emphasizing the importance of breaking down complex problems into manageable steps. Students are encouraged to carefully read and analyze the problem statement, identifying key information such as known variables and what needs to be solved. Visual aids such as diagrams or Punnett squares are recommended to help visualize genetic crosses and predict outcomes.
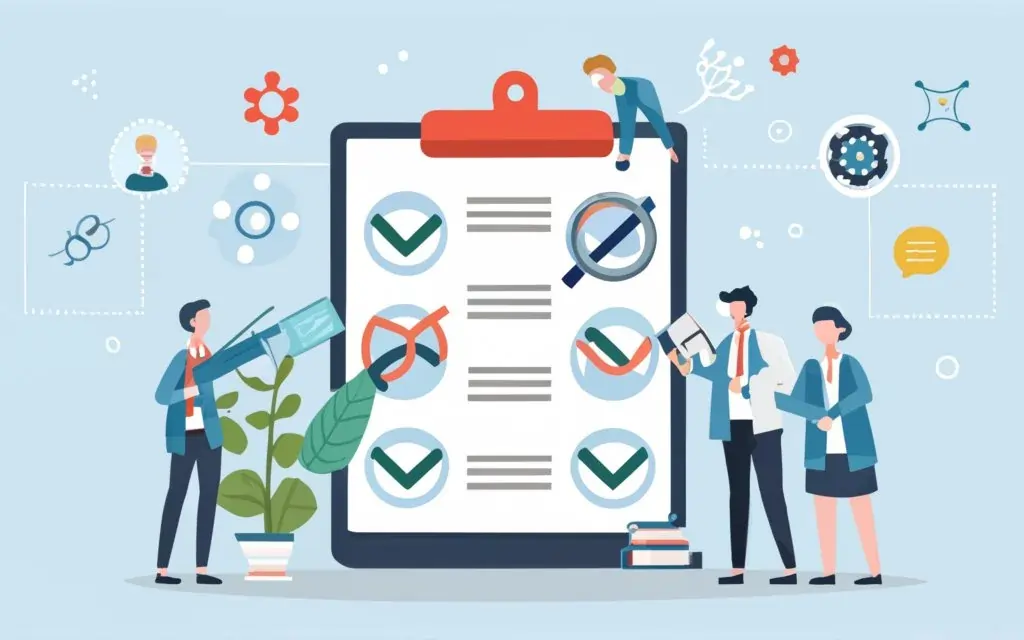
The application of genetic principles is central to solving genetics problems effectively. The blog covers various scenarios where these principles can be applied, including predicting outcomes of monohybrid or dihybrid crosses, calculating probabilities using Punnett squares, and analyzing pedigrees to trace the inheritance of genetic disorders. Concepts such as incomplete dominance, codominance, and sex-linked inheritance are also discussed, expanding the toolkit available to students for solving genetics problems across different contexts.
In addition to understanding concepts and problem-solving strategies, the blog emphasizes the importance of utilizing available resources. Textbooks, online resources, and academic support services are highlighted as valuable tools for enhancing learning and problem-solving skills. Online tools and simulations are particularly recommended for providing interactive experiences that facilitate exploration of complex genetic phenomena.
Regular practice and review are identified as essential components of mastering genetics problem-solving. Students are encouraged to engage in practice problems, both assigned and supplementary, to reinforce concepts and identify areas for improvement. Reviewing completed assignments and seeking feedback from instructors or peers are also recommended strategies for enhancing problem-solving skills and deepening understanding.
Understanding the Fundamentals of Genetics
Understanding the Fundamentals of Genetics offers a concise yet comprehensive overview of the foundational principles that underpin the study of genetics. This brief description serves as a primer for those seeking to grasp the essential concepts within the field.
The exploration begins with an elucidation of Mendelian inheritance, elucidating how traits are passed from one generation to the next according to specific patterns outlined by Gregor Mendel. These patterns, including the principles of segregation and independent assortment, lay the groundwork for comprehending genetic inheritance in various organisms.
Furthermore, the description delves into the structure and function of DNA, the molecule that carries genetic information. It elucidates how DNA is organized into genes, segments that encode specific traits, and how these genes are located on chromosomes within the cell nucleus. Understanding the role of DNA in protein synthesis and the relationship between genotype and phenotype is essential in comprehending the genetic basis of traits.
The description touches upon the concept of genetic variation, highlighting mechanisms such as mutations, genetic recombination, and genetic drift that contribute to diversity within populations. It underscores the importance of genetic variation in evolution and adaptation, showcasing its relevance in fields ranging from agriculture to medicine.
Moreover, the description addresses the principles of molecular genetics, including gene expression, regulation, and genetic engineering. It elucidates how genes are transcribed into RNA and translated into proteins, underscoring the intricacies of gene regulation that govern cellular processes. The description also introduces the concept of genetic engineering, showcasing how manipulation of DNA enables the creation of genetically modified organisms with desired traits.
Furthermore, the description explores the inheritance of complex traits beyond Mendelian genetics, including polygenic traits, multifactorial inheritance, and the influence of environmental factors. It underscores the interplay between genetic and environmental factors in shaping phenotypic traits, emphasizing the complexity of gene-environment interactions.
Introduction to Genetics
Genetics is a captivating branch of biology that investigates the inheritance of traits across generations. It provides a foundational overview of the fundamental concepts and principles that form the basis of the field of genetics. This brief description serves as an introductory exploration into the fascinating world of genetics and its significance in understanding the inheritance of traits and variation in living organisms.
The exploration begins by elucidating the concept of genetics, which is the study of heredity and the variation of inherited traits in organisms. Genetics encompasses a wide range of topics, including the transmission of traits from one generation to the next, the structure and function of DNA, the role of genes in determining traits, and the mechanisms of genetic variation.
One of the key concepts introduced in an introduction to genetics is Mendelian inheritance, which is based on the principles discovered by Gregor Mendel in the 19th century. Mendel's experiments with pea plants laid the groundwork for our understanding of how traits are inherited through discrete units called genes. Mendelian inheritance patterns, such as dominant and recessive traits, provide a basic framework for understanding genetic inheritance in various organisms.
The description also delves into the structure and function of DNA, the molecule that carries genetic information in all living organisms. DNA is composed of nucleotides arranged in a double helix structure, and it encodes the instructions for building and functioning of an organism. Understanding the structure of DNA and its role in genetic inheritance is essential for comprehending how traits are passed from parents to offspring.
An introduction to genetics explores the concept of gene expression, which is the process by which information encoded in DNA is used to direct the synthesis of proteins. Gene expression plays a crucial role in determining an organism's traits and functions, as different genes are activated or repressed in response to various internal and external factors.
The description also touches upon the concept of genetic variation, which refers to the differences in DNA sequences among individuals within a population. Genetic variation arises from mechanisms such as mutations, genetic recombination, and gene flow, and it is essential for evolution and adaptation in living organisms.
-
Mendelian Inheritance:
Gregor Mendel's groundbreaking experiments in the mid-19th century laid the foundation for our understanding of how traits are inherited. Through his meticulous observations, Mendel formulated principles such as dominance, segregation, and independent assortment, which are fundamental to unraveling genetic puzzles. Grasping these principles is paramount for effectively tackling genetics assignments.
-
Genetic Variation:
Genetic variation encompasses the diversity of DNA sequences among individuals within a population. It serves as the raw material upon which natural selection acts, driving evolution. Delving into genetic variation not only illuminates the multitude of traits present within a species but also elucidates their patterns of inheritance, thus serving as a cornerstone for genetics problem-solving.
-
Genetic Disorders:
Genetic disorders arise from abnormalities in an individual's DNA sequence. These disorders can be inherited from one or both parents or can occur sporadically due to mutations. Studying genetic disorders not only unveils the underlying molecular mechanisms of diseases but also presents avenues for problem-solving in genetic counseling and therapeutic interventions.
-
Population Genetics:
Population genetics explores how genetic variation changes within and between populations over time. It encompasses concepts such as genetic drift, gene flow, and natural selection, which influence the frequency of alleles in a population. Understanding population genetics is essential for addressing questions related to evolutionary biology and conservation genetics.
-
Quantitative Genetics:
Quantitative genetics investigates the inheritance of complex traits influenced by multiple genes and environmental factors. It employs statistical methods to unravel the genetic architecture of traits such as height, weight, and disease susceptibility. Mastery of quantitative genetics is crucial for addressing questions related to heritability, genetic correlations, and breeding programs in agriculture and animal husbandry.
-
Epigenetics:
Epigenetics examines heritable changes in gene expression that occur without alterations to the DNA sequence itself. These changes are influenced by environmental factors and can have profound effects on traits and disease susceptibility. Exploring epigenetics expands our understanding of gene regulation and provides insights into the interplay between nature and nurture in shaping phenotypes.
Tools and Techniques for Genetic Analysis
Tools and Techniques for Genetic Analysis offers a succinct yet insightful exploration of the myriad methods and technologies utilized in the study of genetics. This brief description serves as an introductory overview, providing an understanding of the diverse tools and techniques employed by researchers and scientists in genetic analysis.
The exploration begins with an overview of traditional genetic techniques, including classical Mendelian genetics experiments pioneered by Gregor Mendel. These techniques laid the foundation for understanding basic principles of inheritance and genetic variation, setting the stage for more advanced analyses.
The description then delves into modern molecular techniques that revolutionized genetic analysis, such as polymerase chain reaction (PCR) and DNA sequencing. PCR enables the amplification of specific DNA sequences, facilitating the study of genes and their variations. DNA sequencing technologies, including Sanger sequencing and next-generation sequencing (NGS), allow for the precise determination of DNA sequences, unraveling the genetic code with unprecedented accuracy and speed.
Furthermore, the description explores techniques for genetic mapping and genome analysis, such as linkage mapping and genome-wide association studies (GWAS). Linkage mapping identifies the relative positions of genes on chromosomes, while GWAS examines associations between genetic variations and traits or diseases across the entire genome. These techniques enable researchers to identify genes responsible for specific traits or diseases and understand their underlying genetic architecture.
Moreover, the description addresses tools for gene expression analysis, including microarrays and RNA sequencing (RNA-seq). Microarrays allow for the simultaneous measurement of gene expression levels for thousands of genes, providing insights into gene regulation and cellular processes. RNA-seq offers a high-throughput method for quantifying and analyzing gene expression at the transcriptome level, capturing the dynamic nature of gene expression in various biological contexts.
Additionally, the description highlights techniques for genetic manipulation and genome editing, such as gene cloning and CRISPR-Cas9 technology. Gene cloning involves the insertion of specific DNA sequences into vectors for replication and expression, enabling the study of gene function and regulation. CRISPR-Cas9 technology, a revolutionary genome editing tool, allows for precise modification of DNA sequences, opening new avenues for genetic engineering and gene therapy.
Molecular Techniques in Genetics
Molecular Techniques in Genetics explores the diverse array of laboratory methods and procedures employed to study genetic material at the molecular level. This description provides an overview of the fundamental techniques utilized in genetic research and their applications in various fields.
The exploration begins with an elucidation of DNA isolation and purification techniques. These methods involve extracting DNA from biological samples, such as cells or tissues, and purifying it to remove contaminants. Techniques such as phenol-chloroform extraction, silica-based column purification, and magnetic bead-based purification are commonly used to isolate high-quality DNA for downstream applications.
Furthermore, the description delves into DNA amplification techniques, which are essential for generating large quantities of DNA from limited starting material. Polymerase chain reaction (PCR) is the most widely used DNA amplification technique, allowing for the selective amplification of specific DNA sequences. Variations of PCR, such as reverse transcription PCR (RT-PCR) and quantitative PCR (qPCR), enable the amplification and quantification of RNA and DNA, respectively.
Moreover, the description addresses DNA sequencing methods, which are employed to determine the precise order of nucleotides in a DNA molecule. Sanger sequencing, the traditional method of DNA sequencing, involves chain termination using dideoxynucleotides and gel electrophoresis to separate DNA fragments. Next-generation sequencing (NGS) technologies, such as Illumina sequencing and Oxford Nanopore sequencing, enable high-throughput sequencing of DNA with unprecedented speed and accuracy.
Additionally, the description explores techniques for studying gene expression and regulation. Reverse transcription PCR (RT-PCR), RNA sequencing (RNA-seq), and microarray analysis are commonly used methods for quantifying and profiling gene expression levels. Chromatin immunoprecipitation (ChIP) assays and reporter gene assays allow researchers to investigate the regulation of gene expression at the chromatin and transcriptional levels.
Furthermore, the description addresses gene editing techniques, such as CRISPR-Cas9, which enable precise modifications of DNA sequences in living organisms. CRISPR-Cas9 has revolutionized genetic engineering by providing a versatile and efficient tool for editing genes, creating genetically modified organisms, and studying gene function.
- Polymerase Chain Reaction (PCR):
- DNA Sequencing:
- CRISPR-Cas9 Gene Editing:
- Gene Expression Analysis:
- Genome Editing Tools:
PCR is a cornerstone technique in molecular biology that allows for the amplification of specific DNA sequences. This enables researchers to analyze and manipulate DNA for various applications, including genetic testing, cloning, and sequencing.
DNA sequencing techniques have evolved rapidly, enabling scientists to decipher the entire genetic code of organisms. Next-generation sequencing technologies, such as Illumina and Nanopore sequencing, have made it possible to sequence entire genomes quickly and affordably, opening up new avenues for genetic research and diagnostics.
CRISPR-Cas9 has emerged as a revolutionary tool for editing genes with unprecedented precision. This technology, adapted from a bacterial immune system, allows researchers to make targeted modifications to the genome, including gene knockouts, knock-ins, and base editing. CRISPR-Cas9 has far-reaching implications for genetic engineering, gene therapy, and the study of gene function, offering new approaches to solving genetic problems in diverse fields.
Gene expression analysis techniques, such as RNA sequencing and microarrays, enable researchers to study how genes are turned on or off in different cells and tissues. These techniques provide insights into cellular processes, developmental pathways, and disease mechanisms, facilitating the identification of genes associated with specific traits or diseases.
In addition to CRISPR-Cas9, other genome editing tools, such as TALENs (transcription activator-like effector nucleases) and zinc finger nucleases, have been developed for precise genome engineering. These tools enable researchers to edit genes in a variety of organisms, from bacteria to humans, and hold promise for applications in agriculture, medicine, and biotechnology.
Applying Genetics in Problem-Solving Scenarios
Applying Genetics in Problem-Solving Scenarios" explores the practical application of genetic principles to solve real-world problems and scenarios. This brief description serves as an introduction to the diverse ways in which genetics can be utilized in problem-solving contexts.
The exploration begins by highlighting the role of genetics in various fields, including medicine, agriculture, forensic science, and conservation biology. It elucidates how genetic principles are employed to address challenges and solve problems in these domains, showcasing the versatility and relevance of genetics in diverse contexts.
The description delves into the application of genetics in medical diagnostics and treatment. It discusses how genetic testing and analysis are utilized to diagnose genetic disorders, predict disease risk, and tailor personalized treatment plans based on individual genetic profiles. The description also explores the ethical considerations surrounding genetic testing and the implications for informed decision-making in healthcare.
Additionally, the description explores the application of genetics in forensic science and criminal investigations. It discusses how DNA analysis is used to identify individuals, establish paternity, and link suspects to crime scenes. The description also examines the challenges and limitations of DNA evidence in forensic investigations, including issues related to sample collection, contamination, and interpretation.
Furthermore, the description addresses the role of genetics in conservation biology and biodiversity conservation. It discusses how genetic techniques such as DNA barcoding and population genetics are employed to assess genetic diversity, identify endangered species, and develop conservation strategies. The description also explores the challenges of genetic management in conservation, including genetic drift, inbreeding, and habitat fragmentation.
Case Studies in Genetics Problem Solving
Case Studies in Genetics Problem Solving presents a collection of real-world scenarios where genetic principles are applied to solve specific problems or address complex issues. This brief description provides an overview of how case studies can serve as valuable learning tools in the field of genetics.
Each case study within this collection is designed to illustrate the practical application of genetic concepts and problem-solving strategies in various contexts. These scenarios often involve analyzing genetic data, interpreting pedigrees, predicting inheritance patterns, and devising solutions to genetic-related challenges.
One example of a case study could involve a family with a history of a genetic disorder, such as cystic fibrosis or Huntington's disease. Students would be presented with information about the family's medical history, including affected and unaffected individuals, and tasked with analyzing the inheritance pattern of the disorder within the family tree. Through careful examination of the pedigree and application of Mendelian genetics principles, students can deduce the mode of inheritance and assess the risk of the disorder in future generations. This case study not only reinforces understanding of genetic inheritance patterns but also highlights the importance of genetic counseling and informed decision-making in families affected by hereditary diseases.
Another case study might focus on the application of genetic engineering techniques in agriculture. Students could be presented with a scenario where a crop plant is facing challenges such as susceptibility to pests or environmental stressors. Through the application of genetic engineering principles, students would devise a strategy to introduce genetic modifications that confer resistance to these challenges while maintaining desirable agronomic traits. This case study not only demonstrates the potential of genetic engineering in addressing agricultural issues but also encourages critical thinking and problem-solving skills in designing genetic interventions.
-
Crop Improvement:
Genetic engineering techniques have been instrumental in developing crop varieties with desirable traits such as pest resistance, drought tolerance, and increased nutritional value. By manipulating the genetic makeup of plants, scientists can address challenges related to food security and sustainable agriculture. Case studies in crop improvement highlight the role of genetics in breeding programs and the development of genetically modified organisms (GMOs) for agricultural purposes.
-
Forensic Genetics:
Forensic genetics plays a crucial role in criminal investigations by analyzing DNA evidence to identify suspects, exonerate the innocent, and establish biological relationships. DNA profiling techniques, such as short tandem repeat (STR) analysis and mitochondrial DNA sequencing, help forensic scientists solve crimes and bring perpetrators to justice with a high degree of accuracy. Case studies in forensic genetics demonstrate the use of DNA evidence in solving cold cases, identifying missing persons, and establishing paternity or maternity.
-
Precision Medicine:
Precision medicine utilizes genetic information to tailor medical treatments to individual patients based on their unique genetic makeup. By analyzing genetic variations, doctors can predict a patient's response to specific drugs, leading to more effective and personalized healthcare interventions. Case studies in precision medicine illustrate how genetic testing, pharmacogenomics, and targeted therapies are revolutionizing the diagnosis and treatment of diseases such as cancer, cardiovascular disorders, and rare genetic conditions.
-
Genetic Counseling:
Genetic counseling involves assessing an individual or family's risk of inherited genetic disorders and providing information and support to help them make informed decisions about their healthcare. Genetic counselors use pedigree analysis, genetic testing, and risk assessment tools to guide individuals and families through complex genetic issues. Case studies in genetic counseling showcase the role of genetic counselors in facilitating informed decision-making, addressing psychosocial concerns, and empowering individuals to manage their genetic risks.
-
Conservation Genetics:
Conservation genetics aims to preserve genetic diversity within populations of endangered species and mitigate the effects of inbreeding, genetic drift, and habitat loss. By analyzing genetic data from wild populations, conservation geneticists can design effective management strategies, such as captive breeding programs, habitat restoration, and translocation efforts. Case studies in conservation genetics highlight successful conservation initiatives that have relied on genetic information to safeguard biodiversity and restore threatened species to their natural habitats./
Conclusion:
In conclusion, Genetics Problem Solving: A Step-by-Step Guide for Biology Assignments serves as a comprehensive resource for biology students, providing them with the necessary tools and strategies to navigate the complexities of genetics assignments effectively. By emphasizing foundational concepts, systematic problem-solving approaches, and the application of genetic principles, this guide equips students with the confidence and skills needed to excel in their genetics coursework.
The step-by-step approach outlined in the guide empowers students to break down complex problems, identify key information, and apply relevant genetic principles to arrive at solutions. Through the utilization of visual aids such as diagrams and Punnett squares, students can better visualize genetic crosses and predict outcomes with accuracy. The guide underscores the importance of utilizing available resources such as textbooks, online tools, and academic support services to enhance learning and problem-solving skills. Regular practice and review are emphasized as essential components of mastering genetics problem-solving, allowing students to reinforce concepts and identify areas for improvement.