Understanding DNA Replication and Repair: Key Processes for Molecular Biology Assignments
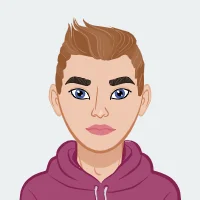
Molecular biology is a fascinating field that delves into the intricate mechanisms governing life at the cellular level. Among the myriad processes within this realm, DNA replication and repair stand out as fundamental pillars. These processes are not only crucial for the continuation of life but also serve as essential subjects for students pursuing molecular biology assignments. In this comprehensive blog, we will unravel the complexities of DNA replication and repair, exploring the key concepts that form the basis of these indispensable molecular mechanisms. The field of molecular biology, a discipline that delves into the intricacies of life at the cellular level, unfolds like a captivating narrative with its central characters being DNA replication and repair. As the very essence of life is encoded in the DNA molecules nestled within our cells, understanding the processes that ensure its faithful transmission and maintenance is crucial. In this blog, we embark on a comprehensive exploration of these fundamental processes, specifically tailored to aid students undertaking molecular biology assignments. DNA replication, akin to a meticulous choreography, unfolds within the cell, guided by the principles of the semiconservative model. If you need assistance with your molecular biology assignment, seeking help from experienced professionals can greatly enhance your understanding and proficiency in DNA replication and repair concepts.
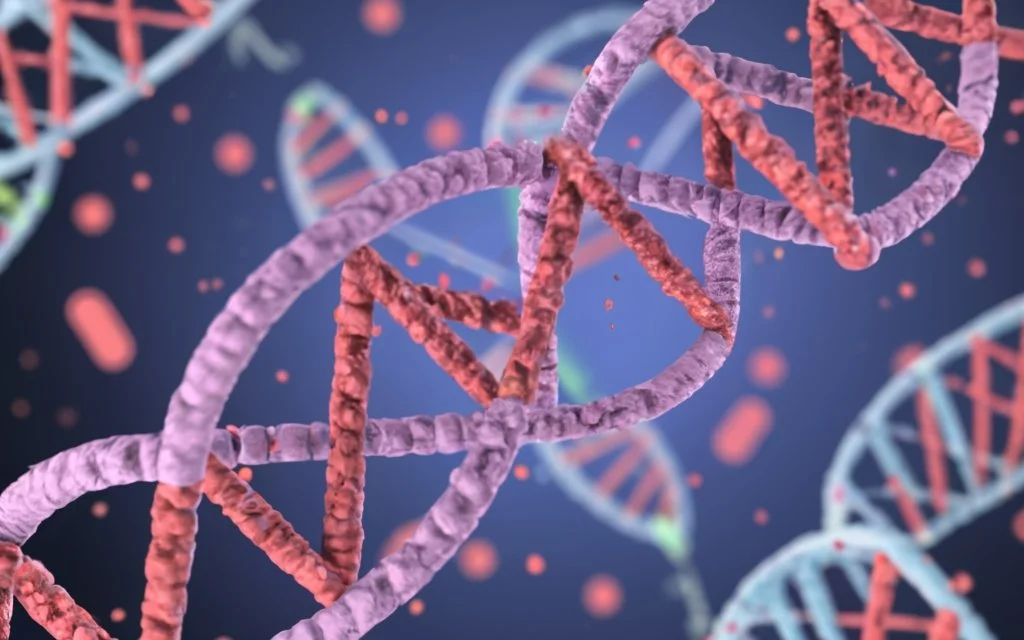
As we unravel this model, we will delve into the intricate molecular details involving DNA polymerases, primase, and helicase, casting light on the synthesis of RNA primers and the elongation of nascent DNA strands. The replication fork, a dynamic structure guiding the DNA replication process, will be dissected to reveal the nuanced interactions between leading and lagging strands, Okazaki fragments, and the sealing action of DNA ligase. Shifting focus to the realm of DNA repair mechanisms, we explore the vigilant guardianship that ensures the integrity of the genetic blueprint. Mismatch repair mechanisms act as proofreaders, diligently identifying and correcting errors that may arise during replication. Nucleotide excision repair, a versatile toolkit, steps in to address damage inflicted by external agents, showcasing the cell's resilience against environmental challenges. This blog aims to be a beacon of knowledge, illuminating the pathways of DNA replication and repair for students navigating the complexities of molecular biology assignments. As we unravel these molecular intricacies, a deeper understanding will blossom, empowering students to appreciate the elegance and precision embedded in the very fabric of life itself. Armed with this knowledge, they will be well-equipped to dissect the mysteries of molecular biology, contributing meaningfully to the ongoing narrative of scientific exploration.
In the vast landscape of molecular biology, the processes of DNA replication and repair emerge as pivotal narratives, essential for deciphering the mysteries of life's blueprint. DNA replication, resembling a meticulous dance of molecules, orchestrates the faithful transmission of genetic information. This blog unfurls the layers of the semiconservative model, revealing the intricate roles played by DNA polymerases, primase, and helicase in the meticulous duplication of DNA. The replication fork, a dynamic stage where leading and lagging strands perform a molecular ballet, takes center stage, highlighting the precision required for the synthesis of new genetic material. Transitioning to the guardians of genetic integrity, the focus shifts to DNA repair mechanisms. Mismatch repair serves as a vigilant proofreader, ensuring the accuracy of the genetic code, while nucleotide excision repair acts as a versatile toolkit, adept at addressing damage caused by environmental assailants. As we navigate through these molecular narratives, students are beckoned into the captivating world of molecular biology, where the intricacies of DNA replication and repair unfold as chapters in the grand story of life. This blog serves as a guiding beacon, illuminating these pathways for students embarking on molecular biology assignments, fostering a profound appreciation for the elegance and complexity inherent in the cellular mechanisms that underpin our existence.
DNA Replication - The Blueprint for Life
DNA replication, often referred to as the blueprint for life, is a remarkable and intricate process that lies at the heart of cellular existence. Envisioned by the seminal work of James Watson and Francis Crick, the semiconservative model serves as the guiding principle for this fundamental biological phenomenon. At its core, DNA replication is the mechanism through which cells ensure the faithful transmission of genetic information from one generation to the next. This process is essential for growth, development, and the perpetuation of life itself.
The semiconservative model of DNA replication unveils the elegant dance of molecules within the cellular nucleus. The DNA double helix, with its complementary base pairs, serves as the template for the synthesis of two identical daughter strands. The orchestration of this symphony involves a cast of molecular players, each with a specific role. DNA polymerases, enzymes with remarkable accuracy, traverse the template strands, adding nucleotides with precision to form the nascent DNA strands. Primase initiates the process by synthesizing short RNA primers, which provide a starting point for DNA polymerases. Helicase unwinds the DNA double helix ahead of the replication fork, creating single-stranded templates ready for replication.
Replication fork dynamics add another layer of complexity to this molecular ballet. As the helicase unwinds the DNA, the leading strand is synthesized continuously in the 5' to 3' direction, matching the direction of the unwinding. The lagging strand, however, is synthesized discontinuously in short fragments called Okazaki fragments. These fragments are later stitched together by the action of DNA ligase, resulting in the completion of both strands.
This process of DNA replication is not only fascinating in its precision but also essential for the perpetuation of genetic information. The fidelity of DNA replication ensures that each daughter cell receives an accurate copy of the genetic material, preserving the integrity of the organism's genetic code. Understanding the molecular intricacies of DNA replication provides a profound insight into the mechanisms that underpin life, making it a central theme in molecular biology. As students delve into this topic for assignments, they embark on a journey into the very essence of cellular existence, gaining appreciation for the complexity and precision inherent in the blueprint for life.
The Semiconservative Model: Unveiling the Blueprint's Secret
At the heart of every living organism lies the miraculous process of DNA replication, a carefully orchestrated dance of molecules that ensures the faithful transmission of genetic information from one generation to the next. The semiconservative model, proposed by James Watson and Francis Crick, is a cornerstone concept in understanding how this process unfolds. The semiconservative model of DNA replication stands as a cornerstone in molecular biology, revealing the elegant secret behind the perpetuation of life's blueprint. Conceived by James Watson and Francis Crick, this model offers profound insights into the faithful transmission of genetic information from one generation to the next. At its core, the term "semiconservative" signifies that each newly replicated DNA molecule consists of one original (parental) strand and one newly synthesized (daughter) strand.
The process commences with the unwinding of the DNA double helix by helicase enzymes, creating a replication fork where the action unfolds. DNA polymerases, the molecular architects of this process, strategically move along the separated strands, reading the nucleotide sequence of the parental DNA, and meticulously assembling complementary nucleotides to form the nascent DNA strand. This synthesis proceeds in the 5' to 3' direction, a critical detail that ensures the precision and fidelity of the replication process.
Primase, another vital player in this intricate molecular orchestra, synthesizes short RNA primers that provide the starting points for DNA polymerases. These primers mark the initiation sites for the synthesis of the new DNA strands. As DNA polymerases extend these primers by adding nucleotides, a continuous leading strand and a discontinuous lagging strand composed of Okazaki fragments are formed.
The significance of the semiconservative model lies in its ability to maintain genetic consistency through successive generations. The resulting DNA molecules, with one strand inherited from the original DNA and the other newly synthesized, carry forward the genetic information encoded in the parent molecule. This meticulous process ensures the accuracy and integrity of the genetic code, playing a pivotal role in the perpetuation of life. As students delve into the intricacies of the semiconservative model, they unlock the secrets of DNA replication, gaining a profound appreciation for the precision and elegance embedded in the blueprint that guides the remarkable journey of life.
Replication Fork Dynamics: Navigating the Molecular Maze
As the replication process kicks into gear, a dynamic structure known as the replication fork emerges, guiding the enzymes and proteins involved in the intricate choreography of DNA replication. This inner topic will delve into the details of replication fork dynamics, exploring the functions of leading and lagging strands, Okazaki fragments, and the essential role of DNA ligase in sealing the gaps. Replication fork dynamics represent a captivating phase in the intricate journey of DNA replication, resembling a molecular maze where precision and coordination are paramount. As the helicase enzymes unwind the DNA double helix, a dynamic structure known as the replication fork emerges, guiding the molecular machinery responsible for synthesizing new DNA strands.
At the heart of this process is the concept of leading and lagging strands. The leading strand is synthesized continuously in the 5' to 3' direction, mirroring the direction of the unwinding DNA. On the other hand, the lagging strand is synthesized discontinuously in short fragments called Okazaki fragments. This asymmetry arises due to the antiparallel nature of DNA strands, requiring a distinct approach for the synthesis of each strand.
The intricacies of replication fork dynamics become apparent as DNA polymerases move along the leading and lagging strands. The leading strand is smoothly elongated as the polymerase continuously adds nucleotides in the direction of unwinding. Meanwhile, the lagging strand encounters a challenge as it must be synthesized in the opposite direction of the fork's movement. This necessitates the synthesis of short RNA primers by primase at regular intervals along the lagging strand. DNA polymerase then adds nucleotides to these primers, forming Okazaki fragments.
The coordination of these events is orchestrated with remarkable precision. DNA ligase, the "molecular glue," joins the Okazaki fragments, creating a seamless, fully synthesized lagging strand. This process ensures that both the leading and lagging strands are synthesized with accuracy and completeness, despite the asymmetrical demands imposed by the antiparallel nature of DNA.
Understanding replication fork dynamics is pivotal not only for comprehending the mechanics of DNA replication but also for appreciating the intricate molecular choreography that safeguards the faithful transmission of genetic information. As students navigate the molecular maze of replication fork dynamics, they unveil the elegance and complexity inherent in the cellular processes that underpin life's remarkable continuity.
DNA Repair Mechanisms - Safeguarding the Blueprint
DNA repair mechanisms emerge as the vigilant guardians of the genetic blueprint, standing as a crucial line of defense against the constant threat of errors and damage. In the intricate dance of life at the cellular level, these mechanisms serve to maintain the integrity of the genetic code, ensuring its accuracy and functionality across successive generations.
Mismatch repair constitutes one key aspect of this safeguarding process. While DNA replication is a highly accurate and intricate affair, occasional errors, or mismatches, may occur. Mismatch repair mechanisms step in as meticulous proofreaders, identifying and correcting these errors to preserve the precision of the genetic code. Proteins such as MutS, MutL, and MutH work in harmony to recognize and excise the mismatched bases, allowing the cell to rectify potential errors before they become permanent alterations in the genetic material.
Nucleotide excision repair, another critical mechanism, addresses a broader spectrum of DNA damage. From environmental factors such as UV radiation to the impact of chemical mutagens, DNA is constantly exposed to potential harm. Nucleotide excision repair serves as a versatile toolkit, capable of recognizing and excising a range of DNA lesions. Endonucleases make incisions around the damaged segment, removing it from the DNA strand. DNA polymerases then step in to synthesize a pristine replacement, and the repaired strand is sealed back into the DNA molecule.
These DNA repair mechanisms underscore the resilience of the cell, constantly working to counteract the challenges posed by both internal and external factors. As students delve into the intricacies of mismatch repair and nucleotide excision repair, they gain insights into the molecular strategies employed by cells to preserve the sanctity of the genetic blueprint. This understanding not only enriches their comprehension of molecular biology but also instills an appreciation for the intricate strategies that life employs to ensure the continuity and fidelity of the genetic code.
Mismatch Repair: Proofreading the Genetic Code
While DNA replication is a highly accurate process, occasional errors, or mismatches, can occur. Mismatch repair mechanisms act as the genome's proofreaders, identifying and correcting these errors to maintain the integrity of the genetic code. Mismatch repair serves as a meticulous proofreading mechanism within the intricate realm of DNA maintenance, ensuring the fidelity of the genetic code by identifying and rectifying errors that may occur during DNA replication. This essential process stands as a sentinel against the potential consequences of mismatched base pairs, a phenomenon that could otherwise lead to mutations and compromise the integrity of the genetic blueprint.
At its core, mismatch repair is a dynamic and highly orchestrated process involving a series of specialized proteins. The primary actors in this molecular drama are MutS, MutL, and MutH. The process is initiated by MutS, which recognizes and binds to the mismatched base pair, marking it as a site requiring correction. MutS then collaborates with MutL to form a complex that scans along the DNA strand, verifying its integrity.
The coordination reaches a critical point with the involvement of MutH. This protein identifies the newly synthesized strand by recognizing a specific DNA sequence, often known as a hemimethylated site. The hemimethylated site indicates that the strand is the result of recent DNA replication. Once identified, MutH makes an incision near the mismatch, targeting the error for removal.
The ensuing steps involve the excision of the mismatched section and the subsequent resynthesis of the corrected DNA strand. The gap is filled by DNA polymerase, which synthesizes a new section using the intact, complementary strand as a template. Finally, DNA ligase seals the nicks in the sugar-phosphate backbone, restoring the continuity of the DNA molecule.
Mismatch repair is a testament to the cell's commitment to maintaining the precision of the genetic code. As students delve into the intricacies of this process, they gain a profound appreciation for the molecular choreography that underlies the proofreading mechanisms within our cells, safeguarding the accuracy and fidelity of the genetic information that dictates the very essence of life.
Nucleotide Excision Repair: Cutting Out the Imperfections
Beyond mismatches, DNA is constantly exposed to various damaging agents, including UV radiation and chemical mutagens. Nucleotide excision repair serves as the cell's repair toolkit for addressing a wide array of DNA lesions. Nucleotide excision repair (NER) stands as a versatile and indispensable mechanism within the cellular toolkit, specifically designed to address a broad spectrum of DNA damage. This process serves as a vigilant guardian against the potential imperfections inflicted upon the genetic code by various environmental factors, such as exposure to ultraviolet (UV) radiation, chemical mutagens, and other sources of genomic stress.
At its essence, nucleotide excision repair is akin to a surgical procedure at the molecular level, wherein damaged segments of DNA are precisely excised and replaced. The process commences with the recognition of the damaged region by a complex of proteins that include XPA, XPC, and TFIIH. This intricate molecular machinery scans the DNA, identifying distortions in its structure caused by lesions or chemical modifications.
Once the damaged site is pinpointed, endonucleases make incisions on both sides of the lesion, effectively cutting out the imperfect segment. This excision creates a gap in the DNA strand, leaving room for repair synthesis. DNA polymerases then step in to synthesize a new, undamaged strand using the intact complementary strand as a template. The synthesis proceeds until the gap is completely filled.
The final step involves sealing the repaired segment back into the DNA double helix. DNA ligase acts as the molecular glue, catalyzing the formation of phosphodiester bonds and restoring the structural and functional integrity of the DNA molecule.
Nucleotide excision repair showcases the cell's adaptability and resilience in the face of genomic challenges. By effectively cutting out and replacing damaged segments, this repair mechanism ensures that the genetic code remains unblemished despite the onslaught of environmental factors. As students delve into the intricacies of nucleotide excision repair, they unravel the sophisticated strategies cells employ to maintain the stability and fidelity of the genetic blueprint, further enriching their understanding of the molecular dynamics that govern life at the cellular level.
Conclusion:
As students venture into the realm of molecular biology assignments, a solid understanding of DNA replication and repair becomes paramount. The intricacies of the semiconservative model, replication fork dynamics, mismatch repair, and nucleotide excision repair collectively weave the tapestry of molecular processes that underpin life itself. By delving into these topics, aspiring molecular biologists can gain a profound appreciation for the elegance and precision embedded in the very fabric of existence. Armed with this knowledge, they are better equipped to unravel the mysteries of life at the molecular level and contribute to the ever-evolving field of molecular biology. In the intricate dance of molecular biology, where the very essence of life is scripted in the DNA molecules nestled within our cells, the exploration of DNA replication and repair reveals a captivating narrative. As we conclude our journey into the realms of the semiconservative model, replication fork dynamics, mismatch repair, and nucleotide excision repair, a profound appreciation for the precision and complexity inherent in these processes blossoms.
The semiconservative model, conceived by the visionaries Watson and Crick, unravels the secret behind life's blueprint, demonstrating how each new generation inherits a faithful copy of the genetic code. Replication fork dynamics, akin to a molecular maze, showcase the remarkable coordination required for the synthesis of leading and lagging strands, revealing the intricacies of the DNA replication process.
DNA repair mechanisms, the vigilant guardians of the genetic blueprint, emerge as heroes in the cellular narrative. Mismatch repair acts as a meticulous proofreader, correcting errors that may arise during replication, while nucleotide excision repair functions as a versatile toolkit, adept at excising and replacing damaged DNA segments caused by environmental factors.
As students navigating the complexities of molecular biology assignments, this exploration provides more than academic insight. It offers a glimpse into the intricacies of life's choreography at the cellular level, where precision, coordination, and resilience converge to safeguard the sanctity of the genetic code. The semiconservative model and DNA repair mechanisms underscore the elegance embedded in the very fabric of existence.
In the grand tapestry of molecular biology, the understanding of DNA replication and repair becomes a compass guiding future molecular biologists in their pursuit of unraveling life's mysteries. Armed with this knowledge, students are not only equipped to dissect the intricacies of the molecular ballet but are also poised to contribute meaningfully to the ongoing narrative of scientific exploration. As we conclude this exploration, we recognize that the journey into the molecular intricacies of life is not just an academic pursuit but a voyage into the profound beauty of the molecular mechanisms that govern our existence.