CRISPR-Cas9 Technology: Revolutionizing Molecular Biology
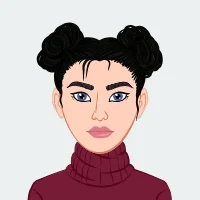
In the dynamic realm of molecular biology, one technological breakthrough stands out as a game-changer: CRISPR-Cas9. This revolutionary tool has not only transformed the landscape of genetic research but has also opened up unprecedented possibilities in fields ranging from medicine to agriculture. If you need assistance with your molecular biology assignment, understanding the profound impact of CRISPR-Cas9 provides valuable insight into the cutting-edge advancements shaping the field of molecular biology.
CRISPR-Cas9, an acronym for Clustered Regularly Interspaced Short Palindromic Repeats and CRISPR-associated protein 9, represents a powerful gene-editing system derived from the natural defense mechanisms of bacteria and archaea. Originally discovered as part of the bacterial immune system, scientists have harnessed its potential to precisely edit DNA in a variety of organisms, including humans.
At the heart of CRISPR-Cas9 technology lies the ability to target specific genes with remarkable accuracy. The system consists of two key components: the guide RNA (gRNA) and the Cas9 protein. The gRNA is designed to match the DNA sequence of the target gene, guiding the Cas9 protein to the precise location in the genome. Once at the target site, Cas9 acts like molecular scissors, cutting the DNA at that specific point. This cut triggers the cell's natural repair mechanisms, allowing for the introduction of new genetic material or the removal of existing sequences.
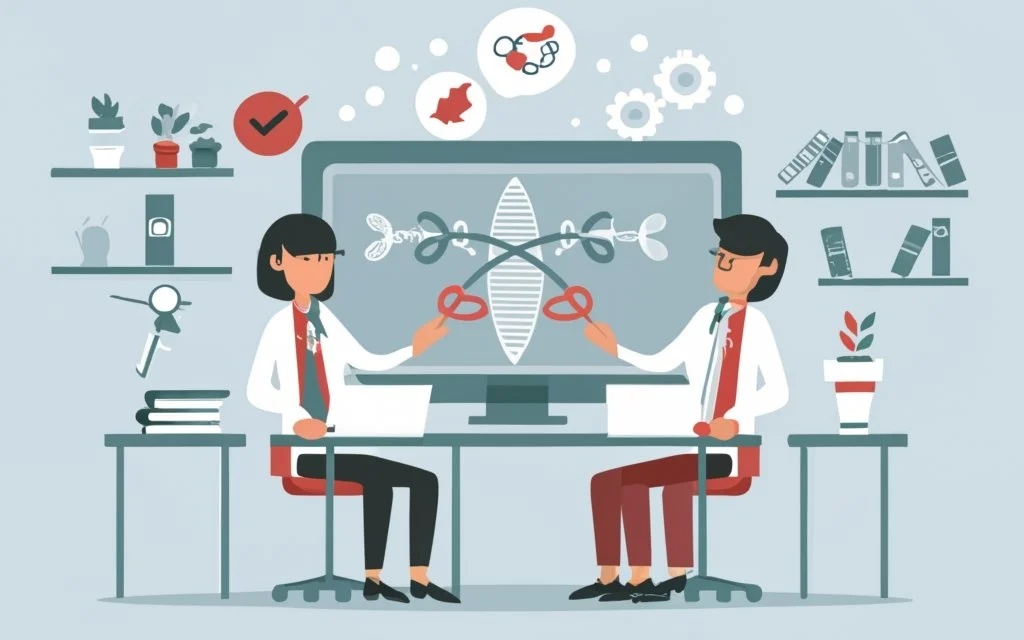
The simplicity and versatility of CRISPR-Cas9 have sparked a revolution in molecular biology research. Previously, gene editing was a complex and time-consuming process, often involving less precise methods. CRISPR-Cas9 has streamlined the process, enabling researchers to modify genes with unprecedented speed and accuracy. This efficiency has significantly accelerated the pace of scientific discovery, allowing scientists to investigate the function of specific genes, understand genetic diseases, and explore potential therapeutic interventions.
In medicine, CRISPR-Cas9 holds immense promise for the treatment of genetic disorders. Researchers are exploring its potential to correct or replace faulty genes responsible for diseases such as cystic fibrosis, muscular dystrophy, and sickle cell anemia. The technology has also been employed in cancer research, with the aim of developing targeted therapies that can selectively eliminate cancerous cells while sparing healthy ones.
Beyond human health, CRISPR-Cas9 has applications in agriculture, environmental science, and biotechnology. In agriculture, researchers are using gene editing to develop crops with enhanced resistance to pests and diseases, improved nutritional content, and increased yields. This has the potential to address global food security challenges and reduce the environmental impact of agriculture. In environmental science, CRISPR-Cas9 is being explored for applications such as environmental cleanup and conservation efforts.
However, with great power comes ethical considerations and challenges. The precision of CRISPR-Cas9 raises concerns about unintended consequences and the potential for "off-target" effects. The ethical implications of editing the human germline – the genetic material passed on to future generations – are particularly significant and have sparked global debates about the responsible use of this technology.
The Genesis of CRISPR-Cas9
The genesis of CRISPR-Cas9 marks a fascinating journey through the annals of microbial defense mechanisms and the convergence of scientific curiosity. This groundbreaking gene-editing technology had its roots in the exploration of bacteria's natural immune systems and has since evolved into a revolutionary tool transforming molecular biology.
The story begins with the discovery of peculiar repetitive DNA sequences in the genomes of bacteria and archaea. These sequences, named Clustered Regularly Interspaced Short Palindromic Repeats (CRISPR), were first identified in the late 1980s by Japanese researchers. However, the significance of CRISPR remained enigmatic until the early 2000s when scientists recognized them as an integral part of the bacterial defense system against viral infections.
Within the CRISPR regions, researchers identified short, unique DNA sequences that matched those of known viruses. This led to the hypothesis that bacteria were using these sequences as a memory system, storing information about past viral encounters. The next key discovery was the identification of CRISPR-associated (Cas) proteins, which played a crucial role in the bacterial immune response.
In 2012, the scientific world was introduced to the revolutionary potential of CRISPR-Cas9 through the collaborative efforts of researchers, including Jennifer Doudna and Emmanuelle Charpentier. Their landmark paper described the use of CRISPR-Cas9 as a programmable gene-editing tool. The Cas9 protein, a molecular scissors guided by a synthetic RNA molecule (guide RNA or gRNA), could be precisely directed to specific DNA sequences, enabling targeted gene modifications.
The simplicity and precision of CRISPR-Cas9 immediately captured the imagination of the scientific community. The technology's potential to edit genes with unparalleled accuracy opened up a myriad of possibilities for genetic research, medical applications, and beyond. It wasn't long before laboratories worldwide adopted CRISPR-Cas9 as the go-to tool for genome editing, replacing older, less efficient methods.
The genesis of CRISPR-Cas9 also spurred a rapid evolution of the technology itself. Researchers began developing variations and improvements, such as the introduction of new Cas proteins with different properties and enhanced precision. These advancements expanded the scope of CRISPR applications, making it a versatile tool for various genetic manipulations.
The impact of CRISPR-Cas9 extends far beyond the laboratory. The technology has triggered a paradigm shift in genetic research, enabling scientists to explore the functions of genes with unprecedented speed and accuracy. Medical research has been particularly influenced, with ongoing efforts to harness CRISPR-Cas9 for the treatment of genetic diseases and the development of personalized therapies.
As the story of CRISPR-Cas9 continues to unfold, challenges and ethical considerations have emerged. The prospect of editing the human germline and the potential for unintended consequences demand careful scrutiny and responsible use. The scientific community and society at large are engaged in ongoing dialogues about the ethical boundaries and regulations surrounding CRISPR technology.
Unraveling the DNA Editing Revolution
The DNA editing revolution represents a groundbreaking era in biotechnology, promising unprecedented control over the genetic code that governs life. At the forefront of this revolution is CRISPR-Cas9, a powerful and precise tool that allows scientists to edit genes with unparalleled accuracy. This revolutionary technology has the potential to transform medicine, agriculture, and various other fields.
CRISPR-Cas9, or Clustered Regularly Interspaced Short Palindromic Repeats and CRISPR-associated protein 9, is a molecular scissors of sorts, enabling scientists to modify DNA sequences with remarkable precision. Its development has paved the way for targeted gene editing, making it possible to add, delete, or replace specific genes within an organism's genome. This has profound implications for treating genetic disorders, as researchers explore the possibility of correcting defective genes responsible for diseases.
One of the key features of CRISPR is its versatility. It has been employed in a multitude of applications, from developing genetically modified crops with enhanced resilience to pests and environmental conditions to creating animal models for studying human diseases. The potential benefits of CRISPR extend to human health, where it holds promise for developing personalized therapies tailored to an individual's genetic makeup.
However, the DNA editing revolution is not without ethical and safety concerns. The ability to manipulate the genetic code raises questions about the unintended consequences of altering the natural order. Ethical considerations come to the forefront when discussing the potential misuse of this technology, such as attempts at creating designer babies with specific traits. Striking a balance between the remarkable potential for positive change and the ethical implications is an ongoing challenge for the scientific community.
Despite these challenges, the DNA editing revolution continues to advance. Researchers are exploring new and improved CRISPR systems, addressing limitations and refining techniques to enhance precision and reduce off-target effects. As the technology progresses, it is likely to play a pivotal role in developing innovative therapies for previously incurable diseases and contributing to advancements in various scientific disciplines.
Mechanisms of CRISPR-Cas9
The mechanisms of CRISPR-Cas9, a revolutionary gene-editing technology, unfold as a captivating interplay between molecular components that mimics the sophisticated defense mechanisms found in bacteria and archaea. Understanding the intricacies of CRISPR-Cas9 requires delving into the roles of its key players and the sequence of events that culminate in precise and targeted gene editing.
At the heart of the CRISPR-Cas9 system is the CRISPR array, a collection of short, partially palindromic DNA sequences interspersed with spacers derived from past viral or plasmid encounters. These spacers serve as a memory bank, allowing bacteria to recognize and mount a defense against specific invaders. The CRISPR array, along with associated Cas proteins, constitutes the adaptive immune system of bacteria.
The CRISPR-Cas9 mechanism involves several crucial steps. The process begins with the transcription of the CRISPR array into a precursor molecule known as pre-CRISPR RNA. This precursor is then processed into shorter RNA molecules called guide RNAs (gRNAs). Each gRNA is designed to complement a specific sequence within the target DNA, guiding the Cas9 protein to the precise location for gene editing.
The Cas9 protein, often referred to as the molecular scissors, is a nuclease that can cut both strands of the DNA double helix. To achieve precision, the gRNA contains a customizable sequence that matches the target DNA. When the gRNA binds to its complementary sequence on the DNA, it directs Cas9 to that specific location, creating a site-specific double-strand break.
Upon reaching the target DNA, the Cas9 protein undergoes a conformational change, forming a structure that allows it to cleave the DNA at the desired location. This cut triggers the cellular repair machinery, initiating one of two repair pathways: non-homologous end joining (NHEJ) or homology-directed repair (HDR).
In the NHEJ pathway, the cell attempts to quickly repair the break by rejoining the DNA strands. This repair process is error-prone and often introduces small insertions or deletions (indels) at the cut site. The introduction of these indels can result in gene knockout or disruption, effectively silencing the targeted gene.
Alternatively, the HDR pathway utilizes a template DNA molecule to repair the cut site with high precision. Researchers can leverage this pathway to introduce specific changes or insert desired genetic material into the target gene. While HDR offers greater control over the editing outcome, its efficiency is often lower compared to NHEJ.
The remarkable precision and versatility of CRISPR-Cas9 have made it a go-to tool for researchers in genetic engineering. However, the technology is not without challenges. Off-target effects, where Cas9 may unintentionally cut DNA at sites similar but not identical to the target, remain a concern. Ongoing research seeks to refine CRISPR-Cas9 and mitigate these off-target effects to enhance its safety and accuracy.
Precision Engineering at the Molecular Level
Understanding the mechanisms behind CRISPR-Cas9 is crucial to appreciating its power and versatility. This section will break down the step-by-step process of CRISPR-mediated gene editing, from the design of the gRNA to the actual modification of the target DNA sequence by the Cas9 protein. The blog will explore how the technology achieves unparalleled precision in its ability to modify specific genes, making it a valuable tool for both basic research and therapeutic applications. Additionally, it will touch upon recent advancements and variations of the CRISPR-Cas9 system that enhance its efficiency and reduce off-target effects. Consider these points:
- Atomic Manipulation: Precision engineering at the molecular level involves the manipulation of individual atoms and molecules, achieved through advanced techniques like scanning tunneling microscopy (STM) and atomic force microscopy (AFM).
- Nanoelectronics Advancements: The field is driving innovations in nanoelectronics, where individual molecules are arranged to form electronic circuits. This promises smaller, more energy-efficient, and potentially faster devices, addressing the limitations of traditional semiconductor technology.
- Medical Applications: Molecular precision engineering is applied in medicine for personalized and targeted therapies. Nanoscale drug delivery systems are designed to transport therapeutic agents directly to specific cells or tissues, minimizing side effects and maximizing treatment efficacy.
- Advanced Diagnostics: Precision engineering enables the development of advanced diagnostic tools capable of detecting diseases at their earliest stages. This has implications for early intervention and more effective medical treatments.
- Materials with Tailored Properties: Materials science benefits from the ability to design materials with specific properties by controlling the arrangement of atoms. This includes the development of lightweight and high-strength materials for aerospace applications and the creation of new catalysts for more efficient chemical processes.
- Revolutionizing Industries: Precision engineering at the molecular level is poised to revolutionize various industries, including electronics, medicine, and materials science. Its impact extends to creating more efficient and innovative solutions in diverse fields.
- Nanotechnology Advancements: The precise control over matter at the molecular scale contributes to the progress of nanotechnology. This includes the development of nanoscale devices and structures with applications ranging from electronics to materials design.
- Energy Efficiency: The precise manipulation of materials at the molecular level can lead to the creation of more energy-efficient devices and systems. This is particularly crucial in the context of sustainable and green technologies.
Applications Across Disciplines
CRISPR-Cas9, with its unparalleled precision in gene editing, has emerged as a transformative technology with applications that span across diverse disciplines. From medicine to agriculture, and from biotechnology to environmental science, the impact of CRISPR-Cas9 reverberates as a versatile tool that holds promise for addressing a myriad of challenges and unlocking new possibilities.
In the realm of medicine, the applications of CRISPR-Cas9 are groundbreaking. Researchers are actively exploring its potential to treat genetic disorders by directly modifying or correcting disease-causing mutations. The ability to target and edit specific genes offers hope for conditions like cystic fibrosis, muscular dystrophy, and sickle cell anemia. Moreover, CRISPR-Cas9 is being harnessed to develop personalized medicine, tailoring treatments to an individual's genetic makeup for more effective and precise interventions.
Cancer research has also witnessed a surge in CRISPR-Cas9 applications. Scientists are leveraging this technology to study the genetic basis of cancer, identify critical genes involved in tumorigenesis, and develop targeted therapies. The specificity of CRISPR-Cas9 allows for the creation of precise models that mimic genetic mutations found in various cancers, facilitating a deeper understanding of the disease and accelerating the development of novel therapeutic approaches.
The agricultural sector has been revolutionized by CRISPR-Cas9, offering innovative solutions to enhance crop yield, nutritional content, and resilience. Researchers are utilizing gene editing to develop crops with increased resistance to pests and diseases, reduced dependence on chemical pesticides, and improved adaptation to environmental stresses. This has significant implications for global food security and sustainable agriculture, addressing the challenges of a growing population and changing climate.
In biotechnology, CRISPR-Cas9 is a game-changer for the production of pharmaceuticals, biofuels, and other valuable compounds. The precise modification of microbial and cellular genomes allows for the optimization of production strains, leading to increased efficiency and yields. This not only accelerates the development of bio-based products but also contributes to the sustainable and cost-effective production of biofuels and other biotechnological applications.
Beyond terrestrial realms, CRISPR-Cas9 is finding applications in environmental science and conservation efforts. Researchers are exploring gene editing to address challenges such as environmental pollution and the conservation of endangered species. The technology's potential for modifying organisms to clean up pollutants or bolster resilience to changing environmental conditions opens up innovative avenues for addressing pressing ecological concerns.
While the applications of CRISPR-Cas9 hold great promise, ethical considerations have emerged, particularly concerning human germline editing. The ability to modify genes that are heritable raises complex ethical, societal, and regulatory questions. Striking a balance between the transformative potential of CRISPR-Cas9 and the responsible use of this technology remains an ongoing challenge that requires careful consideration.
CRISPR-Cas9 Beyond the Petri Dish
CRISPR-Cas9's impact extends far beyond the confines of laboratory experiments. This section will explore the diverse applications of the technology across different fields, including medicine, agriculture, and synthetic biology. From potential cures for genetic disorders to the development of genetically modified crops with enhanced nutritional content, CRISPR-Cas9 is at the forefront of innovation. The inner title will delve into specific case studies and success stories that highlight the real-world implications of CRISPR-Cas9 technology, showcasing its potential to address global challenges and improve human well-being. Consider these points:
- Agricultural Revolution: Beyond the Petri dish, CRISPR-Cas9 is transforming agriculture. It enables precise genetic modifications in crops, enhancing resilience, nutritional content, and yields, addressing challenges like food security and climate change.
- Medical Breakthroughs: In medicine, CRISPR-Cas9 holds promise for treating genetic disorders. Its ability to edit DNA at the molecular level opens avenues for correcting disease-causing mutations, offering hope for previously incurable conditions and even being explored in cancer research.
- Environmental Conservation: Scientists are exploring CRISPR-Cas9 applications in environmental conservation. This includes modifying organisms to adapt to changing climates, potentially mitigating ecological threats and preserving biodiversity.
- Ethical Considerations: The broader applications of CRISPR-Cas9, especially in human germline editing, raise ethical dilemmas. Debates persist regarding the responsible use of this technology and its potential impact on human evolution.
- Global Implications: The technology's impact extends globally, with potential benefits for agriculture, medicine, and environmental sustainability. However, its widespread adoption requires careful consideration of ethical implications and responsible practices.
- Precision and Efficiency: CRISPR-Cas9's precision and efficiency surpass traditional breeding methods, making it acision and Efficiency: CRISPR-Cas9's precision and efficiency surpass traditional breeding methods, making it acision and Efficiency: CRISPR-Cas9's precision and efficiency surpass traditional breeding methods, making it apreferred tool for targeted genetic modifications. This has implications for both research and practical applications.
- Challenges in Implementation: Despite its potential, challenges remain, including off-target effects, unintended consequences, and regulatory frameworks. These factors necessitate ongoing research and careful consideration in implementing CRISPR-Cas9 technologies.
- Uncharted Territory: The journey of CRISPR-Cas9 beyond the Petri dish represents an exploration into uncharted territory. Scientists and policymakers must navigate the evolving landscape of genetic editing technologies with a balance between innovation and ethical responsibility.
Conclusion:
In conclusion, the CRISPR-Cas9 technology stands as a revolutionary force in molecular biology, reshaping the landscape of genetic research and offering unprecedented possibilities across a spectrum of disciplines. Its precision and versatility have propelled advancements in understanding gene function, unraveling the mysteries of genetic diseases, and exploring transformative applications in medicine, agriculture, and environmental science.
The speed and accuracy with which CRISPR-Cas9 enables gene editing have accelerated scientific discovery, allowing researchers to delve into the intricacies of the genome with a precision that was once unimaginable. In medicine, the potential for targeted therapies and the treatment of genetic disorders has opened avenues for personalized medicine, promising more effective and tailored interventions.
The agricultural sector is witnessing a revolution, as CRISPR-Cas9 facilitates the development of genetically modified crops with enhanced traits, addressing global challenges in food security and sustainability. Meanwhile, in biotechnology, the technology's role in optimizing production strains holds promise for the efficient and sustainable production of pharmaceuticals and bio-based products.
However, the transformative power of CRISPR-Cas9 also raises ethical considerations, particularly in the context of human germline editing. The potential to edit heritable genes poses complex ethical dilemmas, necessitating careful consideration and responsible use to ensure the technology's benefits are harnessed without compromising ethical standards.